Inductors are fundamental components in electronics, playing a crucial role in various circuits due to their unique ability to store energy in the form of a magnetic field. Alongside resistors and capacitors, inductors are part of the trio of passive components that form the backbone of electronic circuit design. Inductors are widely used in power supplies, filters, transformers, and signal processing circuits, making them indispensable in both analog and digital electronics.
An inductor is essentially a coil of wire wound around a core, which can be air, iron, or another material. When current flows through the coil, it generates a magnetic field, which in turn induces an electromotive force (EMF) that opposes changes in the current. This property of inductors, known as inductance, allows them to resist changes in current, making them useful for smoothing out power supply fluctuations, filtering noise, and controlling the flow of AC and DC currents.
Inductance is measured in henrys (H), and it depends on factors such as the number of turns in the coil, the core material, and the physical dimensions of the coil. The ability of an inductor to store magnetic energy and oppose changes in current flow makes it a key component in various applications, from basic energy storage to complex signal manipulation in RF circuits.
What is Inductance?
Inductance is the property of an inductor that enables it to store energy in a magnetic field when electrical current passes through it. The inductance L of an inductor is defined by the formula:

where:
- N is the number of turns of the coil,
- μ is the permeability of the core material,
- A is the cross-sectional area of the coil, and
- l is the length of the coil.
Inductance is directly proportional to the number of turns and the permeability of the core material. It is also influenced by the physical dimensions of the coil, such as the diameter and length. The greater the inductance, the stronger the magnetic field generated for a given current, allowing the inductor to more effectively oppose changes in current.
Inductors are characterized by their ability to resist sudden changes in current due to the induced EMF, as described by Faraday’s Law of Electromagnetic Induction. This EMF is given by:
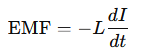
where:
- L is the inductance,
- dI\dt is the rate of change of current.
The negative sign indicates that the induced EMF opposes the change in current, a phenomenon known as Lenz’s Law. This opposition to current changes is why inductors are often used in circuits where it is important to smooth or filter electrical signals.
Types of Inductors
Inductors come in various types, each designed for specific applications based on their construction, core material, and intended use. The most common types of inductors include:
- Air-Core Inductors: These inductors do not use any magnetic core material, relying solely on the air or vacuum inside the coil. Air-core inductors have low inductance values but are free from core saturation and losses, making them ideal for high-frequency applications such as RF circuits, where minimal distortion is critical.
- Iron-Core Inductors: Iron-core inductors use iron or ferrite as the core material to increase inductance by enhancing the magnetic field. These inductors are commonly used in power applications, such as transformers and chokes, where higher inductance values are needed to manage large currents.
- Ferrite-Core Inductors: Ferrite-core inductors use ferrite, a type of ceramic magnetic material, as the core. Ferrites are known for their high permeability and low eddy current losses, making these inductors suitable for high-frequency applications like switching power supplies, filters, and RF circuits. Ferrite cores come in various shapes, including toroidal and pot cores, each offering different performance characteristics.
- Toroidal Inductors: Toroidal inductors are characterized by their doughnut-shaped core, which can be made of iron, ferrite, or powdered metal. The toroidal shape allows the magnetic field to be confined within the core, reducing electromagnetic interference (EMI) and enhancing efficiency. These inductors are widely used in power supplies, audio equipment, and other applications where low noise is essential.
- Choke Inductors: Chokes are inductors specifically designed to block or “choke” high-frequency AC signals while allowing DC or low-frequency currents to pass. They are commonly used in power supply circuits to filter out unwanted noise and ripple, ensuring smooth DC output.
- Multilayer Inductors: Multilayer inductors consist of multiple layers of windings stacked on top of each other, often with a ferrite core. These inductors are compact and provide higher inductance in a smaller form factor, making them suitable for use in miniaturized circuits such as smartphones, tablets, and other portable electronics.
How Inductors Work in Circuits
Inductors function by storing energy in a magnetic field and opposing changes in current. This property makes them useful in a wide range of circuit applications, particularly in power management, filtering, and signal processing.
- Energy Storage: Inductors can store energy in the form of a magnetic field, similar to how capacitors store energy in an electric field. This energy storage capability is utilized in power supplies, where inductors help regulate voltage and current by smoothing out fluctuations. In switching power supplies, inductors store and release energy in a controlled manner, converting input power into a stable output.
- Filtering: Inductors are commonly used in filters to block or allow certain frequencies. In low-pass filters, inductors are used to pass low-frequency signals while attenuating high-frequency noise, making them ideal for audio and RF circuits. In power supplies, inductors are used alongside capacitors to filter out ripple and provide clean DC output.
- Transformers and Coupling: Inductors are integral to the operation of transformers, which use magnetic coupling between primary and secondary windings to transfer energy. Transformers are used to step up or step down voltages in power distribution, isolate circuits, and match impedances in communication systems.
- Chokes and Noise Suppression: Choke inductors are used to suppress high-frequency noise in power lines and signal paths. By impeding high-frequency AC components while allowing DC to pass, chokes help ensure stable operation in electronic devices, reducing electromagnetic interference that can disrupt performance.
- Oscillators and Timing Circuits: Inductors are used in combination with capacitors to create LC (inductor-capacitor) circuits, which form the basis of oscillators and resonant circuits. These oscillators are essential in generating clock signals, RF signals, and other periodic waveforms required in communication and signal processing systems.
- Impedance Matching: In RF circuits, inductors are used for impedance matching, which ensures maximum power transfer between components by matching their impedances. Proper impedance matching is critical in antennas, transmission lines, and amplifiers to optimize performance and minimize signal loss.
Inductors, with their ability to influence current flow through magnetic fields, serve as versatile components in countless applications. Their role in energy storage, filtering, noise suppression, and signal manipulation makes them invaluable in both simple and complex electronic circuits.
In-Depth Look at Inductor Specifications and Ratings
Key Specifications of Inductors
Inductors, like other passive components, are characterized by specific parameters that determine their performance in circuits. Understanding these specifications is essential when selecting an inductor for a particular application, as they directly impact how the inductor will behave under various operating conditions.
- Inductance Value: The inductance value, measured in henrys (H), millihenrys (mH), or microhenrys (µH), indicates the inductor’s ability to store energy in a magnetic field. The value depends on the number of turns in the coil, the core material, and the physical dimensions of the inductor. Common inductance values range from a few nanohenrys (nH) in RF circuits to several henrys (H) in power applications.
- Current Rating: The current rating, also known as the saturation current, specifies the maximum current an inductor can handle before its core saturates. When an inductor’s core saturates, it loses its inductive properties, causing a sharp drop in inductance and potentially damaging the circuit. High current inductors are designed to withstand larger currents without saturating, making them suitable for power applications like DC-DC converters and motor drives.
- DC Resistance (DCR): DC resistance is the resistance of the inductor’s wire windings to direct current. It is measured in ohms (Ω) and contributes to power loss in the form of heat. Lower DCR values are preferred, especially in power circuits, as they minimize energy loss and improve efficiency. However, reducing DCR often requires using thicker wire or more turns, which can increase the size of the inductor.
- Q Factor (Quality Factor): The Q factor is a measure of an inductor’s efficiency, defined as the ratio of its reactance to its resistance at a given frequency. A high Q factor indicates low energy loss and better performance in resonant circuits. Inductors with high Q factors are desirable in RF and high-frequency applications, where minimizing signal attenuation is critical.
- Self-Resonant Frequency (SRF): The self-resonant frequency is the frequency at which the inductor’s inductive and capacitive effects cancel each other out, causing it to behave like a resistor. Above the SRF, the inductor loses its inductive properties and becomes ineffective. Choosing an inductor with an SRF well above the circuit’s operating frequency is essential for maintaining inductive performance.
- Core Material and Saturation: The core material significantly affects an inductor’s performance. Common core materials include air, iron, ferrite, and powdered iron. Each material has different permeability and saturation characteristics. Ferrite cores offer high permeability and are excellent for high-frequency applications, while iron cores provide higher inductance but are more prone to saturation.
- Temperature Coefficient: The temperature coefficient describes how an inductor’s properties change with temperature. Inductors with low temperature coefficients maintain stable performance across varying temperatures, making them suitable for applications in automotive, aerospace, and industrial environments where temperature stability is crucial.
Inductor Markings and Identification
Inductors are often marked with codes or labels that indicate their inductance, current rating, or manufacturer information. While not as standardized as resistor color codes, inductor markings can provide essential clues about the component’s specifications.
- Color Codes: Some inductors, especially older or smaller types, use color bands similar to resistors to indicate their inductance value. However, this practice is less common today, with most inductors marked directly with numerical values.
- Inductance Markings: Inductors are typically labeled with their inductance value followed by a unit abbreviation, such as “10µH” for 10 microhenrys or “1mH” for 1 millihenry. Some small inductors may use shorthand notation, where “102” indicates 1000 µH (1 mH), similar to the capacitor marking system.
- Current and SRF Markings: High-performance inductors often include additional markings for current rating or self-resonant frequency, providing a quick reference for engineers to match the component to circuit requirements.
- Schematic Symbols: In circuit diagrams, inductors are represented by a coiled wire symbol, with variations indicating air-core, iron-core, or variable inductors. Recognizing these symbols helps in understanding circuit functions and identifying the role of each inductor within the system.
Series and Parallel Inductor Configurations
Inductors can be connected in series or parallel configurations to achieve desired inductance values, current ratings, or to manage other circuit characteristics. Understanding how these configurations affect circuit behavior is crucial in designing effective electronic systems.
Series Configuration: In a series configuration, inductors are connected end-to-end, and their inductances add together to create a higher total inductance. This setup is used when a specific, larger inductance value is needed but cannot be achieved with a single inductor. The total inductance Ltotal of inductors in series is given by:

Series configurations also have the benefit of increasing the voltage rating of the combined inductors, which can be advantageous in high-voltage applications.
Parallel Configuration: When inductors are connected in parallel, the total inductance decreases, and the current-handling capacity increases. This configuration allows for distributing current across multiple inductors, reducing the risk of saturation and overheating. The total inductance Ltotal for inductors in parallel is calculated by:

Parallel inductors are often used in power supply circuits and filters where lower inductance and high current capability are needed.
Series-Parallel Combination: Complex circuits may employ both series and parallel combinations of inductors to fine-tune the overall inductance and current capacity. This approach is useful in RF filters, power management systems, and other applications requiring precise control of inductive properties.
Practical Applications of Inductors in Different Fields
Inductors play an essential role across a wide range of applications, each leveraging the unique properties of inductance to achieve specific circuit functions:
- Switching Power Supplies: Inductors are critical components in switching power supplies (SMPS), where they store and transfer energy between input and output stages. By smoothing current flow and filtering noise, inductors help maintain stable voltage and reduce power losses.
- RF and Communication Systems: In RF circuits, inductors are used in tuning, matching networks, and filters to manage signal frequencies and impedance. Inductors, combined with capacitors, form resonant circuits that are the backbone of oscillators, mixers, and RF amplifiers.
- Automotive Applications: Inductors are found in automotive power systems, such as in fuel injection controllers, engine management systems, and electric vehicle power converters. High-current inductors are used to manage power distribution and filter electrical noise in these demanding environments.
- Industrial and Robotics: In industrial and robotic systems, inductors are used in motor drives, inverters, and power conditioners. Their role in managing energy flow, filtering noise, and providing isolation is crucial for maintaining reliable operation in complex machinery.
- Audio Equipment: In audio applications, inductors are used in crossover networks to separate different frequency bands, directing bass, midrange, and treble signals to appropriate speakers. High-quality inductors are essential in hi-fi audio systems to ensure minimal signal distortion.
- Transformers and Energy Transfer: Inductors form the primary and secondary windings of transformers, enabling the transfer of energy between circuits. Transformers are used to step up or step down voltages, provide electrical isolation, and match impedance in power and signal applications.
Inductors, with their diverse configurations and applications, are integral to the design and functionality of countless electronic devices. Their ability to store and manage energy, filter signals, and suppress noise makes them invaluable in both everyday and advanced electronic systems.
Advanced Inductor Applications and Future Trends
Specialized Uses of Inductors
Beyond standard circuit applications, inductors are employed in specialized roles that take advantage of their unique properties. These advanced uses highlight the versatility of inductors in modern technology and their critical importance in various industries.
- Wireless Power Transfer: Inductors are key components in wireless power transfer systems, such as those used in wireless chargers for smartphones, electric toothbrushes, and medical implants. These systems utilize magnetic coupling between coils to transfer energy without physical connections, relying on resonant inductive coupling to efficiently transmit power across short distances.
- Inductive Sensors: Inductors are used in sensors that measure position, speed, or proximity. Inductive proximity sensors, for instance, detect metal objects by measuring changes in inductance caused by the presence of a conductive material. These sensors are widely used in industrial automation, automotive systems, and security devices.
- Magnetic Amplifiers: Magnetic amplifiers, or mag amps, use inductors to control high-power AC signals without moving parts. By adjusting the saturation of the magnetic core, these amplifiers can control large currents efficiently, making them useful in power supplies, motor controllers, and lighting dimmers.
- Inductive Heating: Inductive heating utilizes high-frequency magnetic fields generated by inductors to heat conductive materials. This technique is used in applications such as metal hardening, cooking (e.g., induction stoves), and industrial processing. Inductive heating is favored for its precise control, high efficiency, and ability to target specific areas for heating.
- Energy Harvesting: Inductors play a role in energy harvesting systems, where small amounts of energy are captured from environmental sources like vibrations, heat, or electromagnetic fields. Inductors help convert this harvested energy into usable electrical power, often in conjunction with rectifiers and voltage regulators, for powering low-energy devices like sensors and wearables.
- Magnetic Storage and Memory: Inductors are fundamental to magnetic storage technologies, such as hard drives and magnetic random-access memory (MRAM). In these applications, inductors create magnetic fields that write data to storage media, providing a reliable method for data retention in computing systems.
Challenges and Considerations in Inductor Design
While inductors offer numerous advantages in electronic circuits, they also present unique challenges that must be addressed during the design and selection process.
- Core Saturation and Losses: Core saturation occurs when the magnetic core of an inductor reaches its maximum magnetic flux density, causing a sharp reduction in inductance. This can lead to circuit instability and potential damage. Designers must carefully select core materials and inductor designs to minimize saturation, especially in high-current applications.
- Parasitic Capacitance and Resistance: Inductors inherently possess parasitic capacitance and resistance, which can affect their performance at high frequencies. Parasitic capacitance can create unwanted resonances that alter the expected behavior of the inductor, while parasitic resistance leads to power loss and reduced efficiency. Advanced design techniques, such as minimizing winding capacitance and using high-Q materials, help mitigate these effects.
- EMI and Shielding: Inductors generate magnetic fields that can cause electromagnetic interference (EMI) with nearby components. This is particularly problematic in sensitive circuits, such as RF communication and medical devices. Shielded inductors or ferrite beads are often used to contain magnetic fields and reduce EMI.
- Thermal Management: Inductors, especially those in high-power applications, can generate significant heat due to core and winding losses. Proper thermal management, including heat sinks, airflow, and selecting materials with low core loss, is essential to ensure long-term reliability and performance.
- Size and Form Factor Constraints: The size and form factor of inductors can limit their use in compact devices. Balancing inductance, current rating, and physical size is a challenge, particularly in miniaturized circuits like smartphones and portable electronics. New materials and advanced manufacturing techniques, such as thin-film inductors, are helping address these constraints.
Future Trends in Inductor Technology
Inductor technology is continually evolving to meet the demands of modern electronics. Emerging trends are focused on enhancing performance, reducing size, and improving the efficiency of inductors in cutting-edge applications.
- Miniaturization and High-Frequency Inductors: The trend towards smaller and faster electronic devices drives the need for compact, high-frequency inductors. Innovations in materials, such as nanocrystalline cores and thin-film technologies, are enabling the production of inductors that operate efficiently at high frequencies while occupying minimal space.
- Planar and Integrated Inductors: Planar inductors, which are built directly onto printed circuit boards (PCBs) using flat spiral traces, offer a compact and low-profile alternative to traditional wound inductors. These inductors are particularly useful in power electronics and RF circuits where space and height are constrained. Integrated inductors, often fabricated within ICs, provide even greater integration, reducing the need for discrete components.
- Advanced Core Materials: New core materials, such as amorphous metals and composites, offer improved magnetic properties and lower losses compared to traditional ferrites. These materials enable inductors to operate at higher frequencies with less heat generation, making them suitable for next-generation power converters and energy-efficient designs.
- Flexible and Printed Inductors: The development of flexible electronics has spurred interest in flexible and printed inductors, which can be embedded in wearable devices, smart textiles, and other unconventional applications. These inductors are created using conductive inks on flexible substrates, allowing them to conform to non-traditional shapes and surfaces.
- Environmentally Friendly Inductors: As sustainability becomes a priority, the electronics industry is exploring environmentally friendly inductor designs. This includes using recyclable materials, reducing the use of hazardous substances, and optimizing designs for energy efficiency to minimize environmental impact.
- Smart Inductors: Research is underway to develop inductors with integrated sensors and control capabilities, allowing them to adjust performance dynamically based on circuit conditions. These smart inductors could enable self-tuning power supplies, adaptive filters, and more efficient energy management systems.
Conclusion
Inductors are versatile and vital components in the world of electronics, providing essential functions that range from energy storage and filtering to noise suppression and signal manipulation. Their ability to influence current flow through magnetic fields makes them indispensable in applications as diverse as power supplies, RF communication, automotive electronics, and industrial automation.
The evolving landscape of inductor technology, driven by innovations in materials, design, and manufacturing, continues to expand the capabilities of these components. As electronic devices become smaller, faster, and more efficient, the demand for advanced inductors will only grow, spurring further advancements that will shape the future of electronics.
Whether used in basic circuit designs or cutting-edge technologies, inductors will remain a cornerstone of electronic design, enabling the next generation of innovations in energy management, communication, and beyond.