Biological engineering combines principles from biology, chemistry, and engineering to develop solutions that impact health care, agriculture, and the environment. The integration of 3D printing into biological engineering has emerged as a transformative approach, enabling the fabrication of complex biological systems, devices, and tools with unprecedented precision and customization. This technology enhances research capabilities, streamlines product development, and paves the way for innovative applications that address critical challenges in medicine, agriculture, and ecological sustainability.
The Emergence of 3D Printing in Biological Engineering
Originally used for prototyping in industrial applications, 3D printing has quickly been adopted across various fields of biological engineering due to its versatility and efficiency. Advances in bioprinting and the development of bio-compatible materials have expanded the use of 3D printing to include the creation of tissue scaffolds, synthetic organs, and complex bioreactor designs. This technology’s ability to produce customized and intricate structures makes it an invaluable tool in biological research and application.
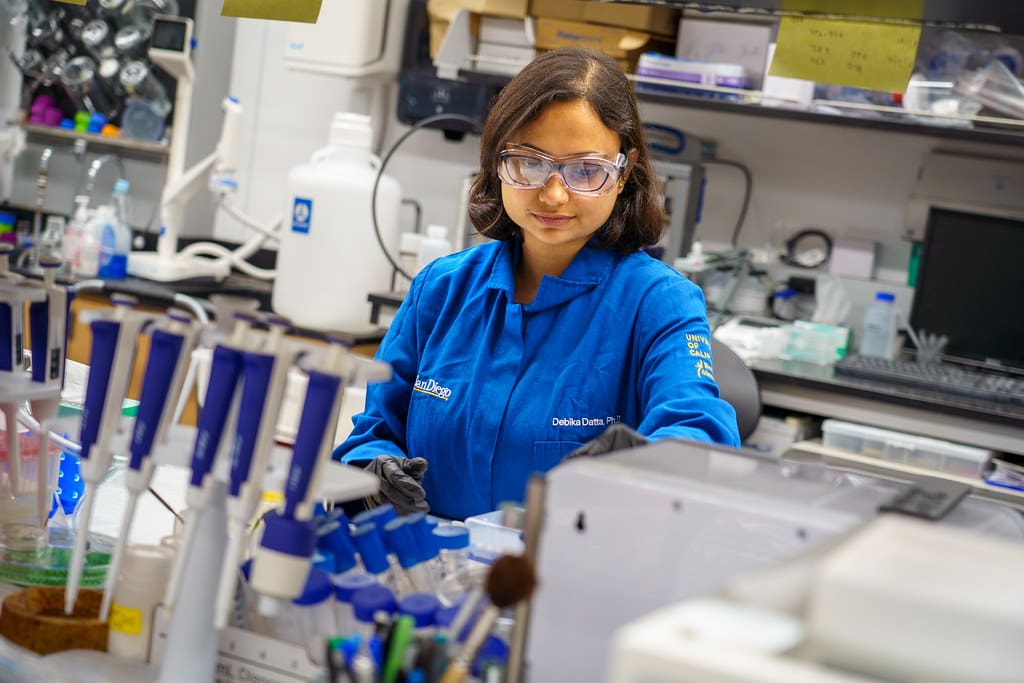
Advantages of 3D Printing in Biological Engineering
Customization and Flexibility: 3D printing allows for the tailored fabrication of biological structures and devices, enabling researchers and engineers to design constructs that meet specific experimental or clinical needs.
Complexity and Scalability: With 3D printing, it is possible to create structures with complex internal architectures that are often required for the growth of biological tissues or the development of specialized bioreactors. The technology also offers scalability, from small-scale laboratory prototypes to larger production models.
Speed and Cost-Efficiency: 3D printing accelerates the design and development process, reducing both the time and costs associated with traditional manufacturing methods. It allows for rapid prototyping, testing, and modification of designs at a fraction of the cost of conventional techniques.
Interdisciplinary Innovation: 3D printing fosters interdisciplinary collaboration by enabling the physical realization of concepts from different fields, facilitating the integration of engineering, biological science, and material science.
Key Applications of 3D Printing in Biological Engineering
Tissue Engineering and Regenerative Medicine: Bioprinting is used to create tissue scaffolds that can support the growth of cells in three dimensions, mimicking natural tissue environments. This application is crucial for developing regenerative medicine therapies, including skin regeneration and complex organ fabrication.
Synthetic Biology: In synthetic biology, 3D printing helps construct microenvironments and bioreactors that can house genetically engineered cells for the production of pharmaceuticals, biofuels, and other biochemically active compounds.
Agricultural Innovations: 3D printing is applied in agriculture to develop plant scaffolds and systems that optimize growth conditions and increase crop yields. It can also be used to create customized tools for precision agriculture.
Environmental Engineering: Biologically engineered devices created through 3D printing, such as biofilters or bioremediation systems, are used for environmental monitoring and cleanup. These systems can be tailored to specific pollutants or environmental conditions.
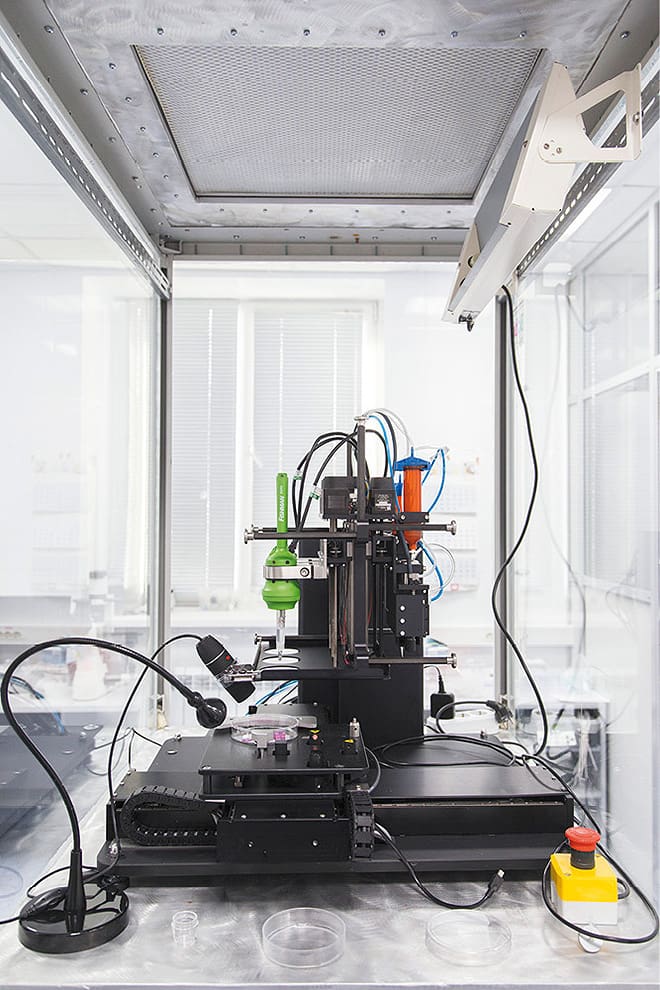
Challenges in 3D Printing for Biological Engineering
Material Limitations: Finding materials that are both printable and biologically functional remains a significant challenge. These materials must often meet stringent standards for biocompatibility, durability, and functionality.
Regulatory and Safety Issues: The use of 3D printed biological devices and systems, especially those intended for clinical or environmental applications, faces regulatory challenges. Ensuring safety, efficacy, and compliance with international standards is critical.
Technical Complexity: Designing and printing functional biological systems requires sophisticated software and hardware, as well as deep understanding of both biological and engineering principles. The complexity of these systems often necessitates extensive collaboration across multiple disciplines.
Ethical Considerations: The ability to print biological materials and systems raises ethical questions, particularly regarding the use and manipulation of living organisms and the potential impacts on natural ecosystems.
Future Directions in 3D Printing for Biological Engineering
The future of 3D printing in biological engineering is promising, with ongoing research and technological advancements expected to overcome existing barriers. Innovations in printable biomaterials, multi-material printing capabilities, and enhanced design software are likely to broaden the scope and impact of 3D printed biological systems. As these technologies mature, they will enable more sophisticated, efficient, and sustainable applications across the spectrum of biological engineering.
3D printing is set to continue its transformative impact on biological engineering, providing innovative tools that enhance the design and implementation of biological systems. As the technology advances, it promises to revolutionize fields ranging from medicine to environmental management, offering more personalized, effective, and sustainable solutions that address some of the most pressing challenges in life sciences.