Optoelectronic components are crucial in modern electronics, enabling devices to interact with light for a variety of applications such as communication, sensing, and display technologies. These components operate on the principle of converting electrical energy into light or vice versa, bridging the gap between optical and electronic systems.
This article delves into the basics of three essential optoelectronic components: Light Emitting Diodes (LEDs), Photodiodes, and Phototransistors. We’ll explore their construction, working principles, and practical applications, highlighting their importance in advancing technologies like IoT, telecommunications, and smart systems.
What Are Optoelectronic Components?
Optoelectronic components are electronic devices that detect or emit light. They are widely used in industries such as automotive, healthcare, and consumer electronics, and their applications include:
- Converting electrical signals into optical signals (e.g., LEDs for illumination).
- Converting optical signals into electrical signals (e.g., photodiodes for light sensing).
- Enabling optical communication systems and sensors.
Optoelectronic devices can be categorized into two main types:
- Light-Emitting Devices: Generate light, such as LEDs and lasers.
- Light-Sensing Devices: Detect and respond to light, such as photodiodes and phototransistors.
1. Light Emitting Diodes (LEDs)
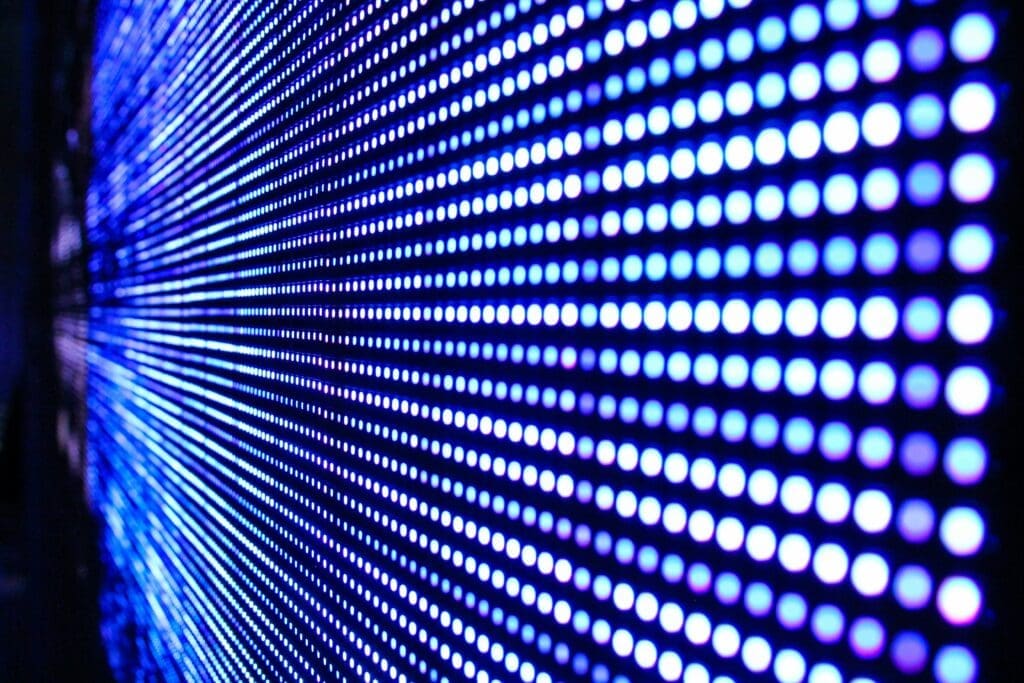
What is an LED?
A Light Emitting Diode (LED) is a semiconductor device that emits light when an electric current flows through it. LEDs are widely used in displays, indicators, and illumination systems due to their energy efficiency, long lifespan, and compact size.
Construction and Materials
- Semiconductor Structure: An LED consists of a p-n junction made of semiconductor materials like gallium arsenide (GaAs) or gallium nitride (GaN).
- Encapsulation: The semiconductor die is encapsulated in a plastic or epoxy lens to focus and protect the emitted light.
- Color Control: The color of light emitted depends on the energy gap (EgE_gEg​) of the semiconductor material.
Working Principle
When a forward bias is applied to the LED:
- Electron-Hole Recombination: Electrons from the n-region combine with holes in the p-region at the junction.
- Photon Emission: This recombination releases energy in the form of photons, producing light.
- Wavelength Dependence: The wavelength (color) of the emitted light depends on the bandgap energy of the material.
Advantages of LEDs
- High energy efficiency compared to incandescent or fluorescent bulbs.
- Long operational life, typically exceeding 50,000 hours.
- Compact size and robustness, suitable for integration into various devices.
- Instantaneous response, ideal for high-speed applications.
Applications of LEDs
- Illumination: Used in homes, streetlights, and vehicle headlights.
- Displays: Integral to TV screens, digital signage, and smartphones.
- Indicators: Found in appliances and electronic devices to signal status or operation.
- Communication: Key components in fiber optic communication systems.
2. Photodiodes
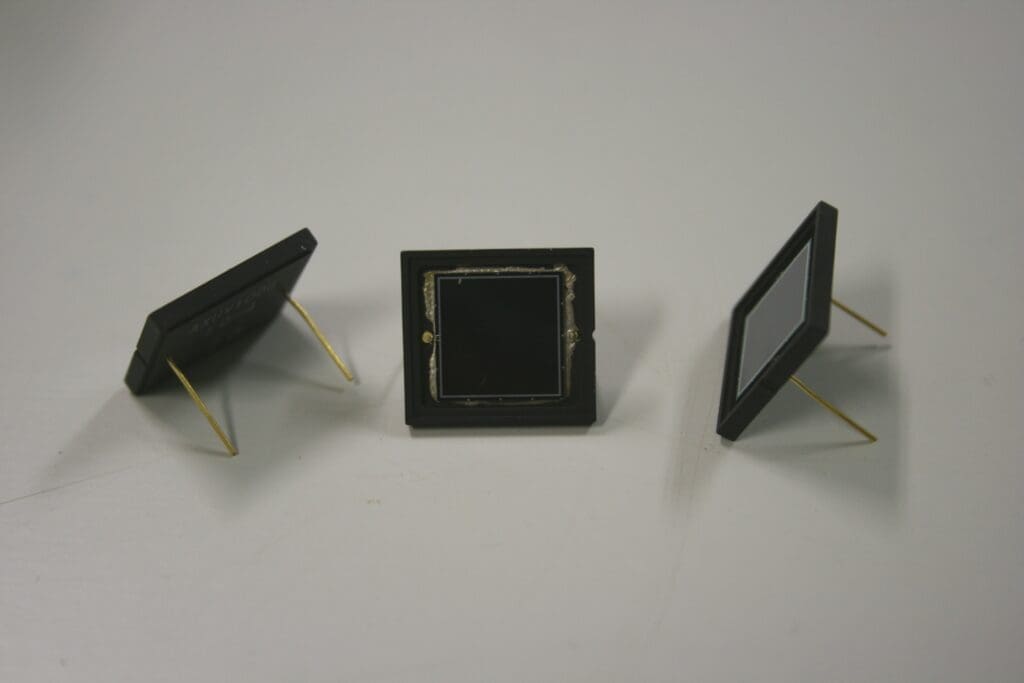
What is a Photodiode?
A photodiode is a semiconductor device that converts light into electrical current. It operates on the principle of the photovoltaic effect, making it a vital component in light detection systems.
Construction and Materials
- Semiconductor Structure: Comprises a p-n junction made of materials like silicon or germanium.
- Package Design: Often housed in a transparent package to allow light to reach the active region.
- Reverse Bias: Operates in reverse bias mode to enhance sensitivity to light.
Working Principle
When light strikes the photodiode:
- Photon Absorption: Photons with sufficient energy excite electrons, creating electron-hole pairs.
- Current Generation: These charge carriers are separated by the electric field at the p-n junction, generating a photocurrent proportional to the light intensity.
Advantages of Photodiodes
- High sensitivity to light, enabling accurate detection of faint signals.
- Fast response time, suitable for high-speed communication systems.
- Linear relationship between light intensity and current, allowing precise measurements.
Applications of Photodiodes
- Light Sensors: Used in cameras, solar cells, and industrial sensors.
- Communication: Detects light signals in fiber optic systems.
- Medical Devices: Integral to pulse oximeters and phototherapy equipment.
- Safety Systems: Deployed in smoke detectors and proximity sensors.
3. Phototransistors
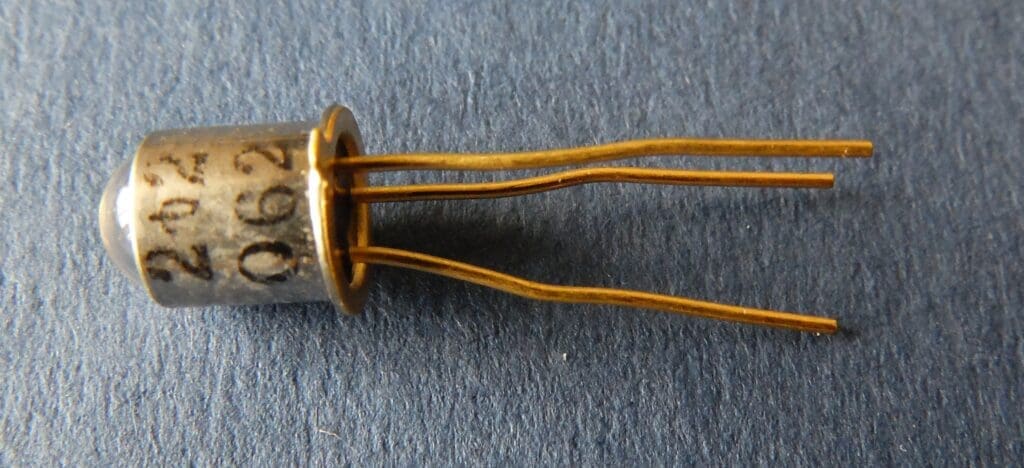
What is a Phototransistor?
A phototransistor is an optoelectronic device that combines the light sensitivity of a photodiode with the amplification properties of a transistor. It is used for applications requiring amplified light detection.
Construction and Materials
- Transistor Base: The base region is light-sensitive and acts as the photodiode.
- Amplification Mechanism: The current generated by the photodiode is amplified by the transistor structure.
- Encapsulation: Packaged in transparent or lens-fitted casings for optimal light exposure.
Working Principle
- Light Activation: When light strikes the base region, it generates a photocurrent due to the photovoltaic effect.
- Current Amplification: The photocurrent is amplified by the transistor, producing a significantly larger output current.
Advantages of Phototransistors
- High sensitivity and amplification, ideal for low-light conditions.
- Simple circuit integration, often replacing separate photodiode and amplifier circuits.
- Cost-effective for applications requiring basic light sensing with amplification.
Applications of Phototransistors
- Optical Switches: Used in automatic lighting systems and door sensors.
- Data Transmission: Key components in infrared receivers for remote controls.
- Industrial Automation: Enable light-based object detection in manufacturing lines.
- Consumer Electronics: Found in optical encoders and ambient light sensors.
Comparison of LEDs, Photodiodes, and Phototransistors
Feature | LED | Photodiode | Phototransistor |
---|---|---|---|
Function | Emits light | Detects light | Detects and amplifies light |
Operation | Forward-biased | Reverse-biased | Light-sensitive base |
Speed | Fast response | Very fast response | Moderate response |
Application | Illumination, displays | Sensors, communication | Amplified light detection |
Design Considerations for Optoelectronic Components
The performance of optoelectronic components depends heavily on their design. Each component must be optimized for its intended application to ensure maximum efficiency and reliability.
1. LEDs: Optimizing Light Output and Efficiency
LEDs are widely used for their energy efficiency, but achieving optimal performance requires careful attention to design parameters.
Key Design Factors:
- Material Selection: The wavelength (color) of light emitted by an LED is determined by the semiconductor material. For example:
- Gallium arsenide (GaAs) for infrared LEDs.
- Gallium phosphide (GaP) for green and yellow LEDs.
- Gallium nitride (GaN) for blue and white LEDs.
- Heat Dissipation: Excess heat can degrade LED performance and lifespan. Effective thermal management, such as using heat sinks, is critical.
- Luminous Efficiency: The efficiency of converting electrical energy into light depends on minimizing energy losses in the p-n junction.
Example Application: In automotive headlights, high-power LEDs are paired with advanced optics and thermal management to produce bright, focused beams while maintaining efficiency.
2. Photodiodes: Enhancing Sensitivity and Response
Photodiodes are essential for applications requiring precise light detection. Optimizing their performance involves several considerations.
Key Design Factors:
- Spectral Response: The sensitivity of a photodiode varies with wavelength. Silicon photodiodes are effective for visible light, while germanium or InGaAs photodiodes are used for infrared detection.
- Quantum Efficiency (QE): QE measures the proportion of photons converted into electrons. Higher QE results in better sensitivity.
- Noise Reduction: Factors like dark current (current in the absence of light) and thermal noise must be minimized to enhance signal accuracy.
Example Application: In fiber optic receivers, high-speed photodiodes with low noise levels are used to accurately convert optical signals into electrical data.
3. Phototransistors: Balancing Sensitivity and Speed
Phototransistors amplify detected light signals, making them suitable for low-light conditions. However, their design must balance sensitivity with response time.
Key Design Factors:
- Amplification Factor (β\betaβ): Higher amplification increases sensitivity but may slow down the response.
- Encapsulation Design: Transparent or focused lens packaging ensures that the maximum amount of light reaches the base region.
- Temperature Stability: Phototransistor performance can vary with temperature, so designs often include materials and packaging that mitigate thermal effects.
Example Application: In optical encoders, phototransistors are used to detect precise rotational or linear positions in machinery.
Real-World Examples of Optoelectronic Components
The versatility of LEDs, photodiodes, and phototransistors allows them to serve a wide range of applications. Here are some detailed real-world examples:
1. LEDs in Smart Lighting Systems
Modern smart lighting systems use LEDs to provide customizable, energy-efficient illumination.
Key Features:
- Color Tuning: By combining red, green, and blue LEDs, smart bulbs can produce millions of colors.
- Dimming Control: LEDs can be dimmed efficiently using pulse-width modulation (PWM), which adjusts the duty cycle of the current.
Example Use Case: Smart streetlights equipped with LEDs can automatically adjust brightness based on ambient light or motion detection, reducing energy consumption and light pollution.
2. Photodiodes in Medical Devices
Photodiodes are widely used in healthcare for their high sensitivity and fast response times.
Key Features:
- Pulse Oximeters: Photodiodes detect changes in light absorption by blood, measuring oxygen saturation levels in real time.
- Phototherapy Devices: UV photodiodes monitor the intensity of light used to treat neonatal jaundice or skin conditions.
Example Use Case: In a wearable fitness tracker, photodiodes work in tandem with LEDs to monitor heart rate by detecting blood flow variations in the wrist.
3. Phototransistors in Industrial Automation
Phototransistors are integral to light-based sensing in industrial environments.
Key Features:
- Proximity Sensors: Detect objects by sensing reflected light, triggering actions like stopping a conveyor belt or activating a robotic arm.
- Safety Systems: Used in light curtains to create an invisible safety barrier that shuts down machinery if an object or person interrupts the beam.
Example Use Case: In a packaging line, phototransistors monitor product alignment and trigger corrective actions to maintain consistent quality.
Factors Influencing Efficiency and Reliability
While optoelectronic components are highly effective, their performance can be affected by several factors.
1. Temperature
- High temperatures can increase the leakage current in photodiodes and phototransistors, reducing accuracy.
- LEDs experience reduced luminous efficiency and accelerated degradation under excessive heat.
Solution:
Thermal management strategies, such as heat sinks, thermal pads, and proper ventilation, are essential.
2. Ambient Light Interference
- For photodiodes and phototransistors, ambient light can create noise and reduce detection accuracy.
Solution:
Use optical filters or shielding to block unwanted wavelengths and focus on the target light source.
3. Material Aging
- Prolonged use can degrade the materials in LEDs, reducing light output and color stability.
Solution:
Select high-quality materials and implement protective coatings to extend lifespan.
4. Signal Noise
- Photodiodes and phototransistors can suffer from electrical and thermal noise, especially in low-light conditions.
Solution:
Circuit designs with proper filtering and shielding can minimize noise interference.
Comparison of Performance in Different Conditions
Component | High-Temperature Performance | Low-Light Sensitivity | Fast Response |
---|---|---|---|
LED | Moderate (requires cooling) | Not applicable | Very fast |
Photodiode | High (with noise considerations) | Excellent | Very fast |
Phototransistor | Moderate (amplification varies) | High | Moderate (slower than photodiodes) |
Emerging Trends in Optoelectronic Components
Optoelectronic technologies continue to advance, enabling smaller, faster, and more efficient devices. Here are some of the most significant trends shaping the future of LEDs, photodiodes, and phototransistors:
1. Miniaturization and Integration
The demand for compact and multifunctional devices has driven the miniaturization of optoelectronic components and their integration into complex systems.
Examples:
- Micro-LEDs: These are much smaller than traditional LEDs and are used in next-generation displays for augmented reality (AR) and virtual reality (VR) devices.
- Monolithic Integration: Photodiodes and phototransistors are being integrated directly onto silicon chips for advanced sensor applications, reducing size and cost.
2. Energy Efficiency Enhancements
Energy efficiency is a key focus, especially for applications like smart lighting and IoT devices.
Examples:
- GaN-Based LEDs: Gallium nitride LEDs offer higher energy efficiency and brightness, making them ideal for high-power applications like automotive headlights and stadium lighting.
- Low-Power Photodiodes: Used in battery-operated devices, these photodiodes minimize energy consumption while maintaining sensitivity.
3. Wavelength Customization
Tailoring the wavelength response of optoelectronic components enables new applications across industries.
Examples:
- UV LEDs: Widely used in disinfection systems for water purification and sterilization.
- Infrared Photodiodes and Phototransistors: Critical for remote sensing, security cameras, and night vision systems.
4. Smart Sensors
Smart optoelectronic sensors combine light detection with data processing capabilities, enabling advanced analytics and decision-making.
Examples:
- LIDAR (Light Detection and Ranging): Combines photodiodes and lasers for 3D mapping, used in autonomous vehicles and geospatial applications.
- Ambient Light Sensors: Found in smartphones and IoT devices, these sensors adjust brightness dynamically based on environmental lighting conditions.
Future Applications of Optoelectronic Components
Optoelectronic technologies are poised to transform numerous industries by enabling innovative applications that leverage their unique capabilities.
1. Artificial Intelligence and Machine Learning
Optoelectronic components are being integrated into AI systems to enhance data collection and processing.
Examples:
- Optical Neural Networks: Use photodiodes and LEDs to perform computations at the speed of light, reducing latency in AI models.
- Smart Cameras: Combine phototransistors with AI algorithms for facial recognition, object detection, and real-time analytics.
2. Renewable Energy Systems
Optoelectronic components are driving advancements in solar power and energy-efficient systems.
Examples:
- Solar Photodiodes: Advanced photodiodes are used in solar panels to convert light into electricity more efficiently.
- LED-Based Energy Harvesting: LEDs with dual functionality can emit and absorb light, allowing them to act as mini solar cells in smart devices.
3. Healthcare and Biomedicine
Optoelectronic devices are revolutionizing diagnostics and treatment in the medical field.
Examples:
- Wearable Health Monitors: Use LEDs and photodiodes to measure vital signs like heart rate, oxygen saturation, and blood pressure.
- Photodynamic Therapy: High-intensity LEDs are used to target and destroy cancerous tissues with minimal side effects.
4. Internet of Things (IoT)
Optoelectronics is a cornerstone of IoT technology, enabling connectivity and smart functionality in devices.
Examples:
- Smart Home Devices: Phototransistors in motion detectors and light sensors for automated lighting and security systems.
- Industrial IoT Sensors: Use photodiodes to monitor equipment conditions, improving maintenance and reducing downtime.
Challenges and Opportunities
While optoelectronic components offer incredible potential, they also face challenges that must be addressed to maximize their impact.
1. Material Limitations
- Many components rely on rare or expensive materials like gallium and indium.
- Scaling production to meet demand while reducing costs is a critical challenge.
Opportunities:
Research into alternative materials like perovskites and organic semiconductors could reduce dependency on rare materials.
2. Thermal Management
- Heat generation in high-power LEDs and phototransistors can affect efficiency and longevity.
- Managing thermal output in compact designs remains a technical hurdle.
Opportunities:
Innovative cooling techniques and materials with high thermal conductivity can enhance performance and reliability.
3. Environmental Impact
- The production and disposal of optoelectronic components can contribute to electronic waste.
Opportunities:
Developing recyclable components and adopting eco-friendly manufacturing processes can address these concerns.
Future Outlook for Optoelectronics
The future of optoelectronics is bright, with continuous innovation pushing the boundaries of what these components can achieve. Here are some key advancements to watch for:
1. Quantum Optoelectronics
Quantum technologies are unlocking new possibilities for optoelectronic components, such as quantum photodiodes and quantum-dot LEDs (QD-LEDs).
Applications:
- Ultra-secure quantum communication networks.
- High-resolution displays with quantum dots.
2. Flexible and Wearable Devices
Flexible optoelectronic components are enabling the development of wearable technologies for healthcare, fitness, and entertainment.
Examples:
- Transparent photodiodes embedded in smart glasses for augmented reality.
- Flexible LEDs in wearable displays for real-time health monitoring.
3. Space Exploration
Optoelectronic components are critical for space missions, providing robust performance in extreme environments.
Examples:
- Radiation-hardened LEDs for spacecraft illumination.
- Photodiodes for solar energy harvesting in satellites.
Conclusion
Optoelectronic components like LEDs, photodiodes, and phototransistors are indispensable in modern technology, driving advancements in industries ranging from healthcare and renewable energy to AI and IoT. With their ability to convert electrical energy into light and vice versa, these components are bridging the gap between optical and electronic systems.
As innovations like quantum optoelectronics, flexible devices, and smart sensors emerge, the possibilities for optoelectronics continue to expand. By addressing challenges like material limitations and thermal management, researchers and engineers can unlock even greater potential for these technologies, shaping the future of electronics and beyond.